Regenerating and Restoring Brain Cells in the Aged With Donor Neural Stem Cells
TUESDAY, 12 JULY 2016 / PUBLISHED IN BLOG
Introduction to Brain Plasticity and Aging
The human brain, as it turns out, is far more malleable than we once thought, even adult brains. However, they are subject to age-related diseases and disorders, such as dementia and diminished cognitive function. There is hope that medical science may be able to replace brain cells and restore memory in aging patients thanks to new discoveries in neural stem cell techniques.
New Techniques in Neural Stem Cell Research
Researchers at the Texas A&M Health Science Center College of Medicine recently published new findings in the journal Stem Cells Translational Medicine. These findings suggest a new technique for preparing donor neural stem cells and grafting them into an aged brain can regenerate tissue that has succumbed to structural, chemical, and functional changes, as well as a host of neurocognitive changes that can be attributed to aging.
Key Findings from the Study
The study, titled “Grafted Subventricular Zone Neural Stem Cells Display Robust Engraftment and Similar Differentiation Properties and Form New Neurogenic Niches in the Young and Aged Hippocampus,” was led by Ashok K. Shetty, Ph.D., a professor in the Department of Molecular and Cellular Medicine. Shetty is also the associate director of the Institute for Regenerative Medicine and a research career scientist at the Central Texas Veterans Health Care System.
Focus on the Aged Hippocampus
Shetty and his team at Texas A&M focus on the aged hippocampus, which plays an important role in making new memories and connecting them to emotions. They took healthy donor neural stem cells and implanted them into the hippocampus of an animal model, enabling them to regenerate tissue.
Significance of the Hippocampus
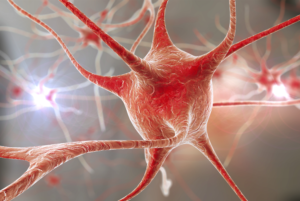
“We chose the hippocampus because it’s so important in learning, memory, and mood function,” Shetty said. “We’re interested in understanding aging in the brain, especially in the hippocampus, which seems particularly vulnerable to age-related changes.” The volume of this part of the brain decreases during the aging process, potentially related to a decline in neurogenesis (production of new neurons) and memory deficits.
Challenges in the Aged Hippocampus
The aged hippocampus exhibits signs of age-related degenerative changes, such as chronic low-grade inflammation and increased reactive oxygen species. Bharathi Hattiangady, assistant professor at the Texas A&M College of Medicine and co-first author of the study, was excited to discover that the aged hippocampus can accept grafted neural stem cells as well as the young hippocampus does, a discovery with significant implications for treating age-related neurodegenerative disorders.
Neural Stem Cell Grafting Process
In this new study, the team found that neural stem cells engrafted well onto the hippocampus in both young and older animal models. Not only did these implanted cells survive, but they also divided several times to create new cells. “They had at least three divisions after transplantation,” Shetty said. “The total yield of graft-derived neurons and glia was much higher than the number of implanted cells, in both the young and aged hippocampus.”
Creation of New Neural Stem Cell Niches
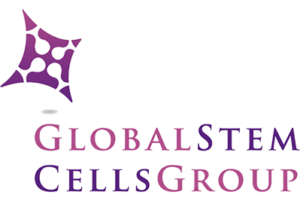
In both old and young brains, a small percentage of the grafted cells retained their stemness feature—an essential characteristic of a stem cell that distinguishes it from ordinary cells—and continuously produced new neurons. This process creates a new ‘niche’ of neural stem cells, which continue to produce new neurons at least three months after implantation.
Comparison to Previous Efforts
Past efforts to rejuvenate brains using fetal neurons were not as successful. Immature cells, such as neural stem cells, can tolerate the hypoxia (lack of oxygen) and trauma of the brain grafting procedure better than relatively mature neurons. The research team used a new technique of preparing the donor neural stem cells, leading to these promising results.
The Role of Brain Marrow
The researchers used donor cells from the sub-ventricular zone of the brain, an area called the “brain marrow,” analogous to bone marrow. This area holds a number of neural stem cells that persist throughout life, continuously producing new neurons that migrate to the olfactory system and respond to injury signals.
Potential of Induced Pluripotent Cells from Skin
Even a small stem cell sample can be expanded in culture, making the procedure minimally invasive. In the future, a single skin cell might suffice to create induced pluripotent stem cells, which can be pushed into neural stem cells. This eliminates the need to obtain cells from the brain, potentially revolutionizing the process.
Future Research Directions
Although the success of the grafted cells is promising, further research is needed to determine if the increased grey matter improves cognition. “Next, we want to test the impact of the implanted cells on behavior and determine if implanting neural stem cells can reverse age-related learning and memory deficits,” Shetty said. He is focused on rejuvenating the aged brain to promote successful aging, maintaining normal cognitive function, and the ability to make memories.
- Published in Blog
Stem Cell-stimulating Fillings Help Regenerate Teeth Damaged by Disease, Decay
MONDAY, 11 JULY 2016 / PUBLISHED IN BLOG
Introduction to Stem Cell-stimulating Fillings
Researchers from Harvard University and the University of Nottingham have developed a new filling that stimulates stem cells in dental pulp to regenerate and even regrow teeth damaged by disease and decay. According to Newsweek Magazine, the discovery earned a prize from the Royal Society of Chemistry after judges described it as a “new paradigm for dental treatments.” The treatment is believed to potentially eliminate the need for root canals.
How the Filling Material Works
The filling works by stimulating the body’s natural store of stem cells to encourage the growth of dentin—the bony material that makes up the majority of the tooth—allowing patients to effectively regrow teeth that are damaged through dental disease. The filling’s synthetic biomaterials are used similarly to dental fillings, placed in direct contact with pulp tissue in the damaged tooth. This stimulates the tissue’s native stem cell population to repair and regenerate pulp tissue and the surrounding dentin.
Comparison to Current Dental Treatments
The discovery is a significant step forward from current methods to treat cavities, which involve drilling out decay and putting in a filling made of gold; porcelain; silver amalgam (which consists of mercury mixed with silver, tin, zinc, and copper); or tooth-colored plastic or composite resin. When these fillings fail to halt the tooth’s decay, a root canal is needed to remove the pulp of the tooth, damaging it even further.
Potential for Industry Adoption
Researchers hope to develop the technique with industry partners to make it available for dental patients as an alternative to traditional fillings. Marie Curie research fellow Adam Celiz says that existing dental fillings are toxic to cells and are therefore incompatible with pulp tissue inside the tooth. “In cases of dental pulp disease and injury, a root canal is typically performed to remove the infected tissues,” Celiz says.
Recognition and Awards
The promise of using therapeutic biomaterials to bring stem cell medicine to restorative dentistry could significantly impact millions of dental patients each year. The approach is so promising it won second prize in the materials category of the Royal Society of Chemistry’s Emerging Technology Competition for 2016. Competition entries were judged on the degree of innovation of the technology, its potential impact, and the quality of the science behind it.
Future of Dentistry with Stem Cell Technology
The stem cell-stimulating filling promises to change the future of dentistry, according to David Mooney, Pinkas Family Professor of Bioengineering at the John Paulson School of Engineering and Applied Sciences at Harvard and the Wyss Institute for Biologically Inspired Engineering. “These materials may provide an effective and practical approach to allow a patient to regenerate components of their own teeth,” Mooney says.
Broader Implications of Stem Cell Research
Stem cells can induce regenerative, self-healing qualities in any tissue found in the body and can, as a result, provide unlimited potential for medical applications. Current studies are underway worldwide to learn how stem cells may be used to prevent or cure diseases and injuries such as Parkinson’s disease, type 1 diabetes, heart disease, spinal cord injury, muscular dystrophy, Alzheimer’s disease, strokes, burns, osteoarthritis, vision and hearing loss, and more. Stem cells may also be used to replace or repair tissue damaged by disease or injury
- Published in Blog
Stem Cell Myths, Busted
THURSDAY, 02 JUNE 2016 / PUBLISHED IN BLOG
Introduction to Stem Cell Myths and Facts
The term stem cell research gleans different reactions from people, both in the medical community and the wider public. Still an emerging science, stem cell research is shrouded by many myths and misconceptions. Here, we take on some of the most predominant myths to discuss the misconceptions and clarify the facts regarding this fast-growing branch of medicine.
Myth #1: Stem Cells Only Come from Embryos
FACT: False. Stem Cells Exist in All Bodies, from Embryos to Adults
Embryonic stem cells come from the early embryo and have the potential to produce all the specialized cells of the body. Because of this, they hold great promise for studying and potentially treating disease and injuries. Tissue or “adult” stem cells are found in the body throughout our lives. These cells maintain and repair many tissues in the body. Examples of these cells include blood stem cells, muscle stem cells, bone marrow stem cells, adipose tissue (fat) stem cells, and skin stem cells. Some of these adult stem cells are used in established medical and aesthetic treatments.
Myth #2: Induced Pluripotent Stem Cells (iPSCs) Eliminate the Need for Embryonic Cells
FACT: False. Research is Needed on All Types of Cells
It is not clear which cells will be most useful for which types of application. For the foreseeable future, side-by-side research on both embryonic and induced pluripotent stem cells is needed. Global Stem Cell Group’s research and treatment products use no embryonic stem cells.
Myth #3: Stem Cell Research Leads to Cloning Humans
FACT: False. Most Countries Prohibit Human Cloning
In most countries, even attempting to clone a human being is illegal. Some countries do allow something called “therapeutic cloning” for the purposes of studying a disease. In this procedure, scientists isolate embryonic stem cells from a cloned blastocyst (early stage embryo) but do not transfer the blastocyst into a womb. These stem cells are genetically matched to the donor organism for studying genetic disease. For example, stem cells could be generated using the nuclear transfer process described above, with the donor adult cell coming from a patient with diabetes or Alzheimer’s. The stem cells could be studied in the laboratory to help researchers understand what goes wrong in diseases like these. Therapeutic cloning also could be used to generate cells that are genetically identical to a patient’s. A patient transplanted with these cells would not suffer the problems associated with transplant rejection. To date, no human embryonic stem cell lines have been derived using therapeutic cloning.
Myth #4: Adult Stem Cells Are Only Found in Adults
FACT: False. Tissue Stem Cells Are Found in People of All Ages
There are three different types of stem cells: embryonic stem cells, induced pluripotent stem cells, and tissue-specific stem cells. It’s the tissue stem cells that are often called “adult” stem cells, but these “adult” stem cells are found in people of all ages.
Myth #5: Embryonic Stem Cell Research Is Banned in Europe
FACT: False. The Laws Vary Across the EU
EU member states have diverging regulatory positions on human embryonic stem cell research. For instance, in Germany, the use of embryos for research is heavily restricted under the Embryo Protection Act (Embryonenschutzgesetz) of 1991, which makes the derivation of embryonic stem cell lines a criminal offense. But in the UK, embryonic stem cell research is allowed, subject to licensing from the Human Fertilization and Embryology Authority (HFEA). Click here for country-by-country overviews for more details. Under the previous two European Framework programs (FP6 and F7), as well as the current program, Horizon 2020, human embryonic stem cell research can be funded, provided that the work is permitted by law in the country where it is to take place.
Myth #6: Stem Cell Research and Treatment Is Against the Law in the US
FACT: False. The FDA Regulates Stem Cell Products but They Are Not Illegal
The FDA does not regulate the practice of medicine, but rather drugs and medical devices and which of these can be marketed in the US. Under federal law, cultured (grown) stem cell products are considered a drug, but are not illegal. Adult stem cells, however, are not cultured—they exist in our bodies throughout our organs, blood, skin, teeth, fat, bone marrow, and other places. Adult stem cell therapy is currently used in the United States to treat conditions such as leukemia and other illnesses. Bone marrow consists of stem cells which have been transplanted for years in the US. Global Stem Cells Group offers stem cell treatments in countries where stem cell therapy is approved and regulated with no appreciable difference in safety record. Stem cell therapy technology is still under review by the FDA.
Myth #7: Bone Marrow Is the Best Source of Stem Cells
FACT: False. Bone Marrow Is Just One Source of Stem Cells
Bone marrow stem cells have been studied for decades and have been used to treat certain types of cancer. A great deal of research has been dedicated to understanding this source of stem cells and their potential. Bone marrow contains a number of different kinds of stem cells, one of which is mesenchymal stem cells. However, mesenchymal stem cells can also be found in adipose (fat) tissue at nearly 2000 times the frequency of bone marrow. Mesenchymal cells have the capability to become different types of tissues (blood vessels, muscle tissue, etc.) and are capable of communicating with other cells. In combination with other proteins, molecules, and regenerative cells found in adipose tissue, they also have the ability to reduce inflammation, regenerate damaged tissue, and grow new blood vessels, a process known as angiogenesis. Stem cells from adipose tissue are more accessible and abundant. They can be processed immediately and reintroduced into the body right away.
Myth #8: There Is a Risk of Rejection with Stem Cell Therapy
FACT: False. Autologous Stem Cells Eliminate the Risk of Rejection
When a patient’s stem cells are derived from his or her own body (such as fat tissue), there is no risk of rejection. In fact, studies thus far have indicated no safety issues with fat-derived autologous (from self) stem cells. Since these stem cells come from your own body, the risk of rejection is eliminated.
- Published in Blog
How Stem Cell Therapies Can Help Heal Sports Injuries
MONDAY, 14 MARCH 2016 / PUBLISHED IN BLOG
Introduction to Stem Cell Therapies in Sports Medicine
Continuing our recent discussion of stem cell therapies for sports injuries, the use of mesenchymal stem cells (MSCs) in orthopedic medicine can help in the repair of damaged tissue by harnessing the healing power of undifferentiated cells that form all other cells in our bodies. The process involves isolating these stem cells from a sample of your blood, bone marrow, or adipose tissue (fat cells), and injecting it into the damaged body part to promote healing. Platelet-rich plasma (PRP), a concentrated suspension of platelets (blood cells that cause clotting of blood) and growth factors, is also used to assist the process of repair.
Cartilage Damage

Cartilage has long been considered an ideal candidate for cell therapy as it is a relatively simple tissue, composed of one cell type, chondrocytes, and does not have a substantial blood supply network. Of particular interest to researchers is the repair of cartilage tissue in the knee, also called the meniscus of the knee. The meniscus is responsible for distributing the body’s weight at the knee joint when there is movement between the upper and lower leg. Only one third of meniscus cartilage has a blood supply, and as the blood supply allows healing factors and stem cells attached to the blood vessels (called perivascular stem cells) to access the damaged site, the meniscus’s natural lack of blood supply impairs healing of this tissue. Damage to this tissue is common in athletes, and is the target for surgery in 60 percent of patients undergoing knee operations, which usually involves the partial or complete removal of the meniscus, which can lead to long-term cartilage degeneration and osteoarthritis.
Recently, researchers have increased their focus on the use of MSCs for treatment of cartilage damage in the knee. Some data from animal models suggest that damaged cartilage undergoes healing more efficiently when MSCs are injected into the injury, and this can be further enhanced if the MSCs are modified to produce growth factors associated with cartilage. It has been shown that once the MSCs are injected into the knee they attach themselves to the site of damage and begin to change into chondrocytes, promoting healing and repair. A small number of completed clinical trials in humans using MSCs to treat cartilage damage have reported some encouraging results, but these studies used very few patients, making it difficult to accurately interpret the results. There are currently a number of ongoing trials using larger groups of patients, and the hope is that these will provide more definite information about the role MSCs play in cartilage repair.
Tendinopathy

Tendinopathy relates to injuries that affect tendons – the long fibrous tissues that connect and transmit force from muscles to bones. Tendons become strained and damaged through repetitive use, making tendinopathy a common injury among athletes. Tendinopathy has been linked to 30 percent of all running-related injuries, and up to 40 percent of tennis players suffer from some form of elbow tendinopathy or “tennis elbow.” Damage occurs to the collagen fibers that make up the tendon, and this damage is repaired by the body through a process of inflammation and production of new fibers that fuse together with the undamaged tissue. However, this natural healing process can take up to a year to resolve, and results in the formation of a scar on the tendon tissue, reducing the tendon’s natural elasticity, decreasing the amount of energy the tissue can store and resulting in a weakening of tendon.
MSCs have the ability to generate cells called tenoblasts that mature into tenocytes. These tenocytes are responsible for producing collagen in tendons. This link between MSCs and collagen is the focus for researchers investigating how stem cells may help treat tendinopathy. Substantial research has been carried out on racehorses as they suffer from high rates of tendinopathy, and the injury is similar to that found in humans. Researchers discovered that by injecting MSCs isolated from an injured horse’s own bone marrow into the damaged tendon, recurrence rates were almost cut in half compared to horses that receive traditional medical management for this type of injury. A later study by the same group showed the MSCs improved repair, resulting in reduced stiffness of the tissue, decreased scarring, and better fusion of the new fibers with the existing, undamaged tendon. It is not yet clear if these results are due to MSCs producing new tenocytes or their ability to modulate the environment around the tendinopathy, as described above. These promising results paved the way for the first pilot study in humans.
Bone Repair

Bones are unique in that they have the ability to regenerate throughout life. Upon injury, such as a fracture, a series of events occur to initiate healing of the damaged bone. Initially, there is inflammation at the site of injury, and a large number of signals are sent out. These signals attract MSCs, which begin to divide and increase their numbers. The MSCs then change into either chondrocytes, the cells responsible for making a type of cartilage scaffold, or osteoblasts, the cells that deposit the proteins and minerals that comprise the hard bone onto the cartilage. Finally, these new structures are altered to restore shape and function to the repaired bone. A number of studies carried out in animals have demonstrated that direct injection or infusing the blood with MSCs can help heal fractures that previously failed to heal naturally. However, as was the case with tendinopathy, it is not yet clear if these external MSCs work by generating more bone-producing cells or through their ability to reduce inflammation and encourage restoration of the blood supply to injured bone, or both.
Brain Injury in Sports

There is mounting evidence that those taking part in sports where they are exposed to repetitive trauma to the head and brain are at a higher risk of developing neurodegenerative disorders, some of which are targets for stem cell treatments. For example, it has been reported that the rate of these diseases, like Alzheimer’s Disease, were almost four times higher in professional American football players compared to the general population. While the cause of this disease is not yet clear, it is associated with abnormal accumulation of proteins in neural cells that eventually undergo cell death and patients develop dementia. Researchers have attempted a number of strategies to investigate treatments of this disease in mice, including introducing neural stem cells that could produce healthy neurons. While some of these experiments have demonstrated positive, if limited, effects, to date there are no stem cell treatments available for Alzheimer’s Disease.
Boxers suffering from dementia pugilistica, a disease thought to result from damage to nerve cells, can also demonstrate some symptoms of Parkinson’s Disease (among others). In healthy brains, specialized nerve cells called dopaminergic neurons produce dopamine, a chemical that transmits signals to the part of the brain responsible for movement. The characteristic tremor and rigidity associated with Parkinson’s Disease is due to the loss of these dopaminergic neurons and the resulting loss of dopamine production. Researchers are able to use stem cells to generate dopaminergic neurons in the lab that are used to study the development and pathology of this disease. While a recent study reported that dopaminergic neurons derived from human embryonic stem cells improved some symptoms of the disease in mice and rats, stem cell-based treatments are still in the development phase.
- Published in Blog